The Supershell-Molecular Cloud Connection:
Large-Scale Stellar Feedback and the Formation of the Molecular ISM
by: J. R. Dawson
[
arXiv:1301.1419 [astro-ph.GA],
pdf,
first author:
personal site]
This is a review paper on most of the current major papers dealing with how molecular clouds form. It is a good reference paper for me to use. A number of the major papers that he cites are ones that I have seen before. She cites five of Fabian Heitsch's papers (my advisor) and a few from Mordecai-Mark Mac Low (Fabian's advisor). So this is a review of many of the papers that are similar to things that I am doing.
The basic purpose of the paper is to review the current state of simulations and observations of molecular clouds in their natural habitat, the ISM. It tries to address the fundamental question, "How, when and where do we form molecular clouds." The problem with molecular clouds is that in order for them to form is that you need sufficient density and column density to shield the cloud from UV radiation that will ionize the cloud and destroy any molecules that form. Any significant heating will also destroy the cloud and prevent it from achieving densities high enough to allow for gravitational collapse.
The current review is focused on how large scale stellar feedback can contribute to the formation and evolution of molecular clouds. In other words how can O and B type stars contribute to an environment that is conducive for the formation and survival of molecular clouds. The idea is that the OB regions will form a super bubble in the galaxy which will form shell walls of gas that has been swept up and compressed by the strong stellar winds. These shells will rapidly cool, and along with hydrodynamics (and magnetohydrodynamics!) and thermal instabilities will cause the shell walls to collapse and form molecular clouds. The interest is in modeling these effects, and also trying to model the interacting flows at the shell boundaries where the molecular clouds actually form.
There are two possible processes for the formation of molecular clouds. The first is through global gravitational collapse of the the galactic disk. This model requires significant inflows of matter from outside the galaxy, usually from the halo or galactic neighborhood. This is where high velocity clouds come in and play a role. Also galactic fountains can be placed in this process, but galactic fountains can also be part of the second process. The second process is from the shocks and turbulence inherent in the galactic disk from gas flows, and star formation. This paper focuses on the second process.
The general idea can be summed up from the first figure in the paper:
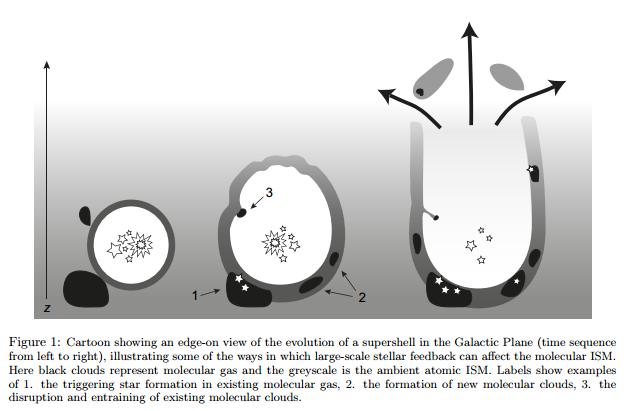
In order to have the blow out as shown in figure 1 there needs to be enough energy from the OB region to push its way out of the galactic disk. There region will sweep up gas from the ISM and will form a shell wall. The question is, what are the characteristics of this wall? And do molecular clouds form there (Section 3), or are they formed elsewhere (Section 4) and are caught up in the wall?
The question of how much energy is needed to cause a blow out depends heavily on the ISM and where the OB region is located and how strong it is. He cites
Mac Low et al. 1989 and
Tenorio-Tagle et al. 1990 (also see
Tenorio-Tagle et al. 1990) on this one. These supershells are defined to have formation energies of E ≥ 10
52 erg. If there is a blow out then the ejected material will supply the halo with metal rich material and more energy.
Here are the section headings and subsection headings for sections 3 and 4, with a brief explanation of what is in the sections. These are the sections that cover modeling and are the sections that I am currently most interested in.
3 Molecular Cloud Formation in Supershells: Theory & Modelling
3.1 Molecule Formation & Destruction
This section covers the conditions that are needed for molecules to survive. We need to know under what conditions molecules can survive in order to constrain our models. This deals with the strength of the UV field that will cause dissociation and ionization.
3.2 Gravitational Instability of Expanding Shells
This section covers the question of at what point will the shell begin to collapse gravitationally. There are conditions that affect whether or not a shell can collapse, such as the strength of the supernovas or OB stars that create the shell. If there is too much energy then the shell will be too hot for collapse. Farther away the shell may begin to collapse, but this is dependent on the ISM and the speed of the shell. Even still there may not be enough time for the shell to collapse before the proto molecular clouds fragment (the fragmentation timescale is shorter than the collapse timescale).
3.3 Molecular Cloud Formation in Colliding Flows
If you have two interacting flows (i.e. two shells raming into each other) then there may be favorable conditions to form molecular clouds. This model relies on thermal instabilities and turbulence to form molecular clouds. The thermal instabilities rely on an interesting property of the pressure density relation of cooling gas. The role of magnetic fields is still being investigated in all of this.
3.4 Whole-Disk Models of the Feedback Structured ISM
These models look at how certain gas structures are formed by having large scale simulations of the entire disk. Dawson includes a figure from
Hill et al. (2012). The third author on the paper is Mordecai-Mark Mac Low. I spoke to Mordecai-Mark last year about this paper when he visited UNC last year. He was able to give me some pointers about how to fix my own problems because they had run into the exact same problems in their simulations. As in I was talking to him and I said, "This is what I am working on, but I am having some problems." and he said, "And you are getting negative temperatures under these conditions. We had the same problem so we hired a math PhD to fix the problem. This is what we did ..."
4 Pre-Existing Molecular Clouds
4.1 Cloud Disruption
This section looks into the conditions associated with the shells and how they interact with a non-homogeneous ISM. This is critical for the survival, growth collapse of pre-existing molecular clouds.
Section 5 looks into observations being made of the Milky Way and of near neighbors (Large and Small Magellanic clouds). By mapping the CO and H
2 in reference to star forming regions we can get a sense of whether or not molecular clouds form inside the shells or form outside the shells and are caught up in them as they sweep by. Also we can look at column densities, structure and expected life span of the clouds. Dawson has done a number of observations of these supershells and her work will be interesting to look into. I may review some of her papers in the future.
Papers Cited:
Hill, A. S., Joung, M. R., Mac Low, M.-M., Benjamin, R. A., Ha ner, L. M., Klingenberg, C., & Waagan, K. 2012, ApJ, 750, 104
Mac Low, M., McCray, R., & Norman, M. L. 1989, ApJ, 337, 141
Tenorio-Tagle, G., Rozyczka, M., & Bodenheimer, P. 1990, A&A, 237, 207